- Volumes 84-95 (2024)
-
Volumes 72-83 (2023)
-
Volume 83
Pages 1-258 (December 2023)
-
Volume 82
Pages 1-204 (November 2023)
-
Volume 81
Pages 1-188 (October 2023)
-
Volume 80
Pages 1-202 (September 2023)
-
Volume 79
Pages 1-172 (August 2023)
-
Volume 78
Pages 1-146 (July 2023)
-
Volume 77
Pages 1-152 (June 2023)
-
Volume 76
Pages 1-176 (May 2023)
-
Volume 75
Pages 1-228 (April 2023)
-
Volume 74
Pages 1-200 (March 2023)
-
Volume 73
Pages 1-138 (February 2023)
-
Volume 72
Pages 1-144 (January 2023)
-
Volume 83
-
Volumes 60-71 (2022)
-
Volume 71
Pages 1-108 (December 2022)
-
Volume 70
Pages 1-106 (November 2022)
-
Volume 69
Pages 1-122 (October 2022)
-
Volume 68
Pages 1-124 (September 2022)
-
Volume 67
Pages 1-102 (August 2022)
-
Volume 66
Pages 1-112 (July 2022)
-
Volume 65
Pages 1-138 (June 2022)
-
Volume 64
Pages 1-186 (May 2022)
-
Volume 63
Pages 1-124 (April 2022)
-
Volume 62
Pages 1-104 (March 2022)
-
Volume 61
Pages 1-120 (February 2022)
-
Volume 60
Pages 1-124 (January 2022)
-
Volume 71
- Volumes 54-59 (2021)
- Volumes 48-53 (2020)
- Volumes 42-47 (2019)
- Volumes 36-41 (2018)
- Volumes 30-35 (2017)
- Volumes 24-29 (2016)
- Volumes 18-23 (2015)
- Volumes 12-17 (2014)
- Volume 11 (2013)
- Volume 10 (2012)
- Volume 9 (2011)
- Volume 8 (2010)
- Volume 7 (2009)
- Volume 6 (2008)
- Volume 5 (2007)
- Volume 4 (2006)
- Volume 3 (2005)
- Volume 2 (2004)
- Volume 1 (2003)
• Meteorological data were examined to determine the long-term trends of fog.
• Vertical structure of atmospheric boundary layer during fog events was analyzed.
• Variation characteristics of air pollutant concentrations during fog events were analyzed.
Long-term trends in fog episodes, vertical variations of atmospheric boundary structure, and air pollutant concentrations during two different heavy fog events in the Tianjin area were analyzed. The total amount of fog has increased since 1980 due to the stability of the boundary layer and an increase of pollutant emissions. The variation in the characteristics of the boundary layer and air pollutant concentrations were significantly different between the two fog processes (fog I and fog II). The onset of fog I was accompanied by a temperature inversion in the low atmosphere, and the average kinetic energy showed a clear diurnal trend and vertical variation, which increased with height. The dissipation of fog I was mainly due to turbulence. However, the atmospheric stratification was not stable in the lower layer before the onset of fog II. The diurnal and vertical changes in kinetic energy were very small, in which turbulent momentum at each measurement height tended to be zero. In the dissipation process of fog II, wind speed increased significantly. Surface PM2.5 concentrations decreased, but the ratio of PM2.5 to PM10 increased from 0.66 to 0.82 until fog I dissipated. However, the concentration of PM2.5 did not decrease at the early stage of fog II, but the ratio of PM2.5 to PM10 PM2.5/PM10 decreased to 0.21 when fog II dissipated. This study showed that there was a clear difference in the evolution of pollutant concentration for different pollutants and in different developing stages during the fog events. PM2.5 concentration accumulated faster than those of SO2 and NOx, and the PM2.5 cumulative rate was greater in the mid-term of the fog process.
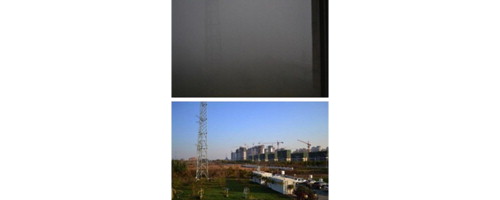