- Volumes 84-95 (2024)
-
Volumes 72-83 (2023)
-
Volume 83
Pages 1-258 (December 2023)
-
Volume 82
Pages 1-204 (November 2023)
-
Volume 81
Pages 1-188 (October 2023)
-
Volume 80
Pages 1-202 (September 2023)
-
Volume 79
Pages 1-172 (August 2023)
-
Volume 78
Pages 1-146 (July 2023)
-
Volume 77
Pages 1-152 (June 2023)
-
Volume 76
Pages 1-176 (May 2023)
-
Volume 75
Pages 1-228 (April 2023)
-
Volume 74
Pages 1-200 (March 2023)
-
Volume 73
Pages 1-138 (February 2023)
-
Volume 72
Pages 1-144 (January 2023)
-
Volume 83
-
Volumes 60-71 (2022)
-
Volume 71
Pages 1-108 (December 2022)
-
Volume 70
Pages 1-106 (November 2022)
-
Volume 69
Pages 1-122 (October 2022)
-
Volume 68
Pages 1-124 (September 2022)
-
Volume 67
Pages 1-102 (August 2022)
-
Volume 66
Pages 1-112 (July 2022)
-
Volume 65
Pages 1-138 (June 2022)
-
Volume 64
Pages 1-186 (May 2022)
-
Volume 63
Pages 1-124 (April 2022)
-
Volume 62
Pages 1-104 (March 2022)
-
Volume 61
Pages 1-120 (February 2022)
-
Volume 60
Pages 1-124 (January 2022)
-
Volume 71
- Volumes 54-59 (2021)
- Volumes 48-53 (2020)
- Volumes 42-47 (2019)
- Volumes 36-41 (2018)
- Volumes 30-35 (2017)
- Volumes 24-29 (2016)
- Volumes 18-23 (2015)
- Volumes 12-17 (2014)
- Volume 11 (2013)
- Volume 10 (2012)
- Volume 9 (2011)
- Volume 8 (2010)
- Volume 7 (2009)
- Volume 6 (2008)
- Volume 5 (2007)
- Volume 4 (2006)
- Volume 3 (2005)
- Volume 2 (2004)
- Volume 1 (2003)
• Evolution of rarefaction wave at the onset of silo discharge was simulated using DEM.
• Shape of the front of rarefaction wave was very sensitive to dimension of discharge orifice.
• Speed of the wavefront ranged from 70 to 80 m/s and of its tail from 20 to 60 m/s.
• DEM correctly reflected rise of rarefaction wave in inter-particle contact network.
The generation of a rarefaction wave at the initiation of discharge from a storage silo is a phenomenon of scientific and practical interest. The effect, sometimes termed the dynamic pressure switch, may create dangerous pulsations of the storage structure. Owing to the nonlinearity, discontinuity, and heterogeneity of granular systems, the mechanism of generation and propagation of stress waves is complex and not yet completely understood.
The present study conducted discrete element simulations to model the formation and propagation of a rarefaction wave in a granular material contained in a silo. Modeling was performed for a flat-bottom cylindrical container with diameter of 0.1 or 0.12 m and height of 0.5 m. The effects of the orifice size and the shape of the initial discharging impulse on the shape and extent of the rarefaction wave were examined. Positions, velocities, and forces of particles were recorded every 10−5 s and used to infer the location of the front of the rarefaction wave and loads on construction members. Discharge through the entire bottom of the bin generates a plane rarefaction wave that may be followed by a compaction wave, depending on the discharge rate. Discharge through the orifice generates a spherical rarefaction wave that, after reflection from the silo wall, travels up the silo as a sequence of rarefaction–compaction cycles with constant wavelength equal to the silo diameter. During the travel of the wave along the bin height, the wave amplitude increases with the distance traveled. Simulations confirmed earlier findings of laboratory and numerical (finite element method) experiments and a theoretical approach, estimating the speed of the front of the rarefaction wave to range from 70 to 80 m/s and the speed of the tail to range from 20 to 60 m/s.
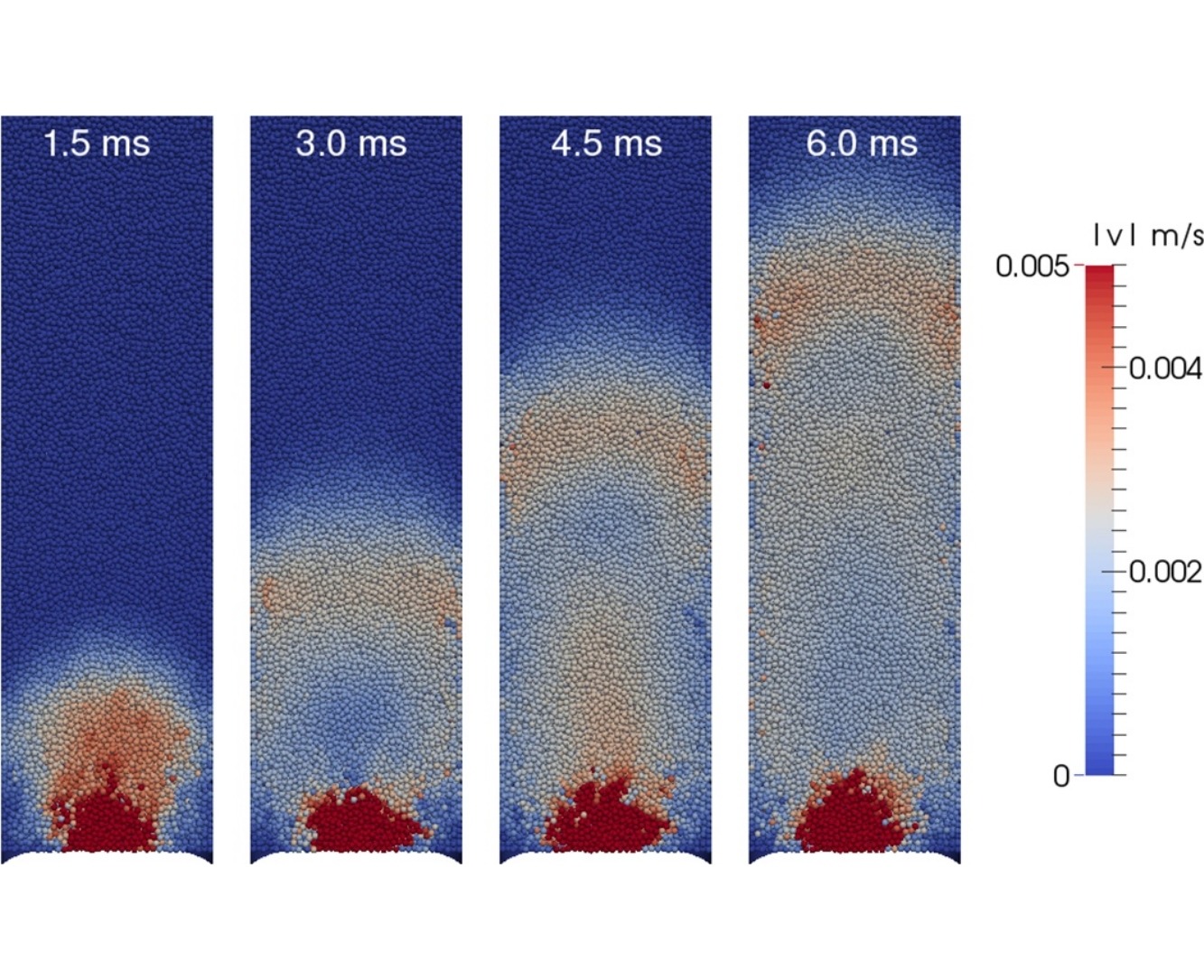