- Volumes 84-95 (2024)
-
Volumes 72-83 (2023)
-
Volume 83
Pages 1-258 (December 2023)
-
Volume 82
Pages 1-204 (November 2023)
-
Volume 81
Pages 1-188 (October 2023)
-
Volume 80
Pages 1-202 (September 2023)
-
Volume 79
Pages 1-172 (August 2023)
-
Volume 78
Pages 1-146 (July 2023)
-
Volume 77
Pages 1-152 (June 2023)
-
Volume 76
Pages 1-176 (May 2023)
-
Volume 75
Pages 1-228 (April 2023)
-
Volume 74
Pages 1-200 (March 2023)
-
Volume 73
Pages 1-138 (February 2023)
-
Volume 72
Pages 1-144 (January 2023)
-
Volume 83
-
Volumes 60-71 (2022)
-
Volume 71
Pages 1-108 (December 2022)
-
Volume 70
Pages 1-106 (November 2022)
-
Volume 69
Pages 1-122 (October 2022)
-
Volume 68
Pages 1-124 (September 2022)
-
Volume 67
Pages 1-102 (August 2022)
-
Volume 66
Pages 1-112 (July 2022)
-
Volume 65
Pages 1-138 (June 2022)
-
Volume 64
Pages 1-186 (May 2022)
-
Volume 63
Pages 1-124 (April 2022)
-
Volume 62
Pages 1-104 (March 2022)
-
Volume 61
Pages 1-120 (February 2022)
-
Volume 60
Pages 1-124 (January 2022)
-
Volume 71
- Volumes 54-59 (2021)
- Volumes 48-53 (2020)
- Volumes 42-47 (2019)
- Volumes 36-41 (2018)
- Volumes 30-35 (2017)
- Volumes 24-29 (2016)
- Volumes 18-23 (2015)
- Volumes 12-17 (2014)
- Volume 11 (2013)
- Volume 10 (2012)
- Volume 9 (2011)
- Volume 8 (2010)
- Volume 7 (2009)
- Volume 6 (2008)
- Volume 5 (2007)
- Volume 4 (2006)
- Volume 3 (2005)
- Volume 2 (2004)
- Volume 1 (2003)
• A microencapsulated phase-change material (MicroPCM) was studied for thermal energy storage.
• Composite MicroPCM particles were prepared using copper particles (CP) and copper foam (CF).
• Thermal conductivities of MicroPCM/CP and MicroPCM/CF were significantly improved.
The application of phase-change materials (PCM) for thermal-energy storage is hampered by their low thermal conductivity. Copper particles (CP) and copper foam (CF) were used to enhance the thermal conductivity of a microencapsulated phase-change material (MicroPCM). The effects of the CP size and mass fraction and the pore number per inch (PPI) of the CF on the thermal properties of the MicroPCM were investigated. The chemical and microstructures of the MicroPCM and its CP composites were characterized by Fourier transform infrared spectroscopy and scanning electron microscopy, respectively. Their thermal conductivities, phase-change temperatures, and latent heats were measured. Adding CP into the MicroPCM decreased the latent heats with increasing loadings. The thermal conductivities of MicroPCM/CP (5 wt%, 50 nm diameter) and MicroPCM/CF (PPI 30) were 1.12 times and 3.46 times that of the unmodified MicroPCM at 20 °C, respectively. Thermal-energy storage performances of the MicroPCM/CF composites were studied at a power of 2.9 ± 0.1 W. The temperature of the contact surfaces of the pure MicroPCM and its composites (PPI 10, PPI 20, PPI 30) was 75.88, 51.27, 50.52, and 50.23 °C, respectively. The composites displayed more uniform temperature distributions.
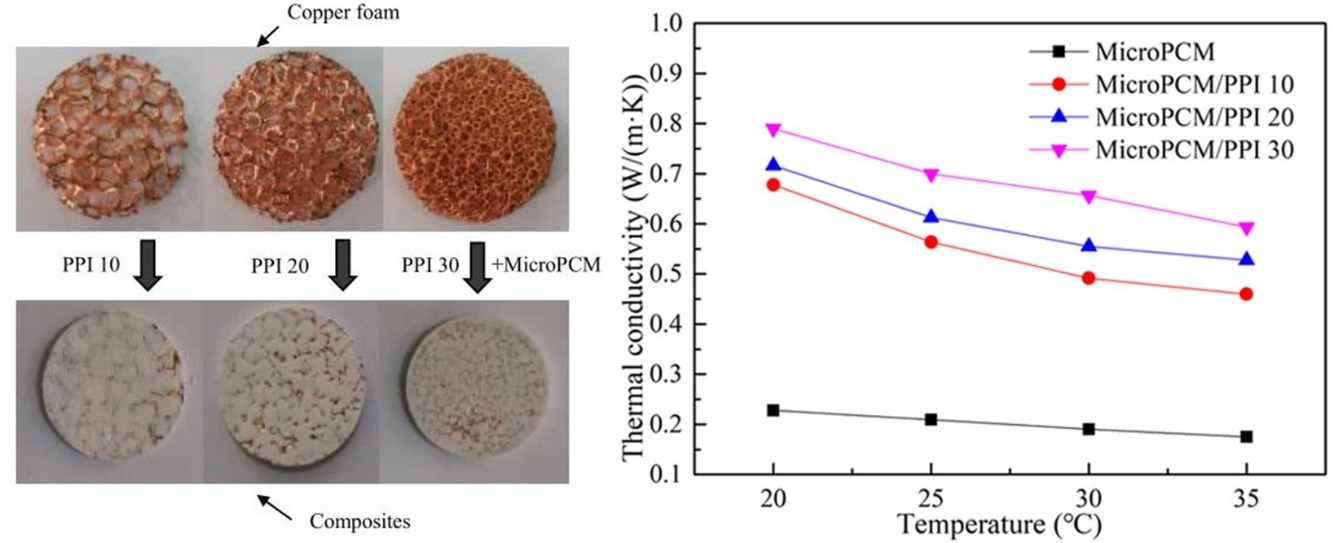