- Volumes 84-95 (2024)
-
Volumes 72-83 (2023)
-
Volume 83
Pages 1-258 (December 2023)
-
Volume 82
Pages 1-204 (November 2023)
-
Volume 81
Pages 1-188 (October 2023)
-
Volume 80
Pages 1-202 (September 2023)
-
Volume 79
Pages 1-172 (August 2023)
-
Volume 78
Pages 1-146 (July 2023)
-
Volume 77
Pages 1-152 (June 2023)
-
Volume 76
Pages 1-176 (May 2023)
-
Volume 75
Pages 1-228 (April 2023)
-
Volume 74
Pages 1-200 (March 2023)
-
Volume 73
Pages 1-138 (February 2023)
-
Volume 72
Pages 1-144 (January 2023)
-
Volume 83
-
Volumes 60-71 (2022)
-
Volume 71
Pages 1-108 (December 2022)
-
Volume 70
Pages 1-106 (November 2022)
-
Volume 69
Pages 1-122 (October 2022)
-
Volume 68
Pages 1-124 (September 2022)
-
Volume 67
Pages 1-102 (August 2022)
-
Volume 66
Pages 1-112 (July 2022)
-
Volume 65
Pages 1-138 (June 2022)
-
Volume 64
Pages 1-186 (May 2022)
-
Volume 63
Pages 1-124 (April 2022)
-
Volume 62
Pages 1-104 (March 2022)
-
Volume 61
Pages 1-120 (February 2022)
-
Volume 60
Pages 1-124 (January 2022)
-
Volume 71
- Volumes 54-59 (2021)
- Volumes 48-53 (2020)
- Volumes 42-47 (2019)
- Volumes 36-41 (2018)
- Volumes 30-35 (2017)
- Volumes 24-29 (2016)
- Volumes 18-23 (2015)
- Volumes 12-17 (2014)
- Volume 11 (2013)
- Volume 10 (2012)
- Volume 9 (2011)
- Volume 8 (2010)
- Volume 7 (2009)
- Volume 6 (2008)
- Volume 5 (2007)
- Volume 4 (2006)
- Volume 3 (2005)
- Volume 2 (2004)
- Volume 1 (2003)
• Weak alkaline nature of NH3·H2O yields unimodal Li2CO3 particle size distributions.
• Supersolubility and secondary nucleation rate decreased as temperature increased.
• The Li2CO3 mean particle size increased as a function of temperature.
• With improved supersaturation the Li2CO3 mean particle size decreased significantly.
• Supersaturation regulates PSDs by influencing secondary nucleation and growth rates.
In this study, the gas‒liquid reactive crystallization of LiCl–NH3·H2O–CO2 was adopted to produce Li2CO3. The weakly alkaline nature of NH3·H2O in the absence of any recarbonation process resulted in a unimodal and easily controllable particle size distribution (PSD) of the obtained Li2CO3. The reaction temperature significantly influenced both the Li2CO3 particle size and PSD. By increasing the temperature from 25 to 60 °C, the volume weighted mean particle size increased from 50.5 to 100.5 μm, respectively. The Li2CO3 secondary nucleation rate and growth rate were obtained by focused beam reflectance measurements and a laser particle size analyzer, respectively. The secondary nucleation rate of Li2CO3 reduced as a function of temperature, whereas the growth rate increased. In addition to improving the surface energy of the crystals to enhance the growth process, higher temperatures also reduced the supersolubility of Li2CO3, which also plays a role to decrease the secondary nucleation rate. At a constant temperature, supersaturation affects the Li2CO3 particle size through the synergistic effect of secondary nucleation and growth. Hence, with improved supersaturation, the mean particle size of Li2CO3 decreased. The results provide a meaningful way to evaluate the crystallization process and to regulate the particle size.
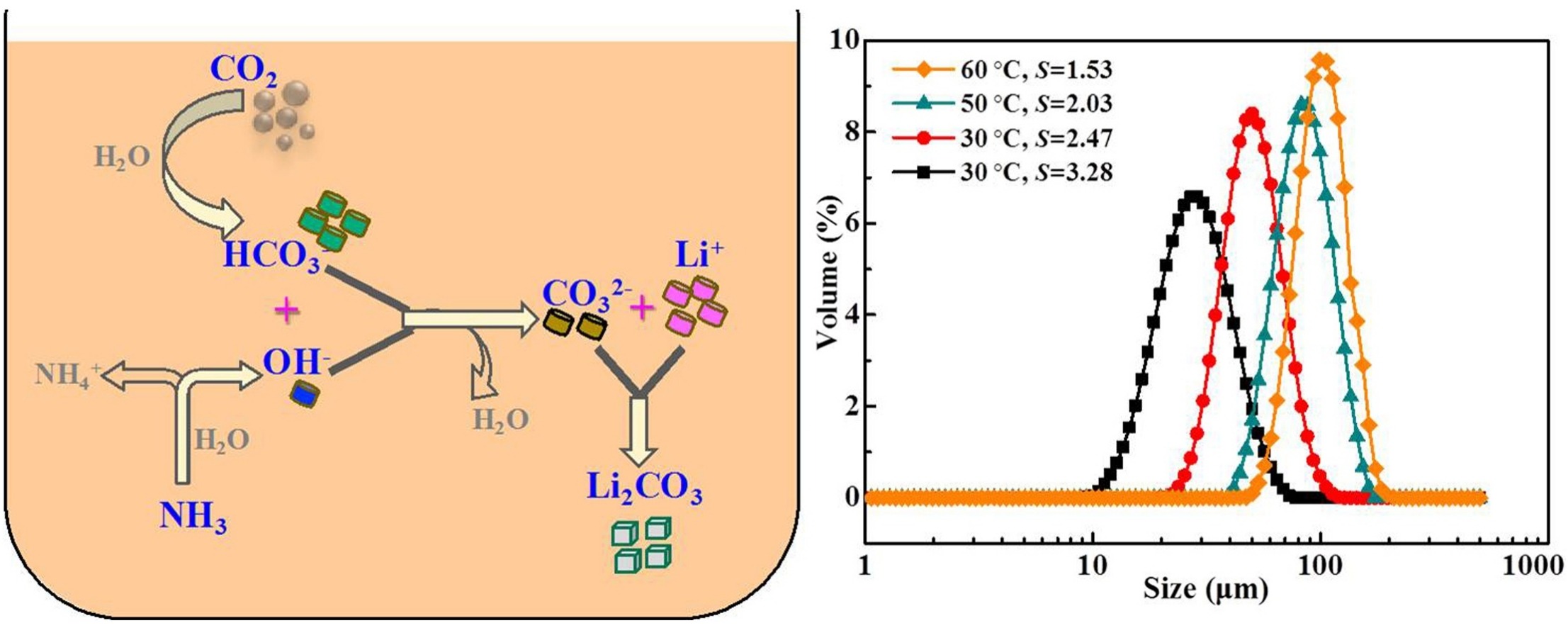