- Volumes 84-95 (2024)
-
Volumes 72-83 (2023)
-
Volume 83
Pages 1-258 (December 2023)
-
Volume 82
Pages 1-204 (November 2023)
-
Volume 81
Pages 1-188 (October 2023)
-
Volume 80
Pages 1-202 (September 2023)
-
Volume 79
Pages 1-172 (August 2023)
-
Volume 78
Pages 1-146 (July 2023)
-
Volume 77
Pages 1-152 (June 2023)
-
Volume 76
Pages 1-176 (May 2023)
-
Volume 75
Pages 1-228 (April 2023)
-
Volume 74
Pages 1-200 (March 2023)
-
Volume 73
Pages 1-138 (February 2023)
-
Volume 72
Pages 1-144 (January 2023)
-
Volume 83
-
Volumes 60-71 (2022)
-
Volume 71
Pages 1-108 (December 2022)
-
Volume 70
Pages 1-106 (November 2022)
-
Volume 69
Pages 1-122 (October 2022)
-
Volume 68
Pages 1-124 (September 2022)
-
Volume 67
Pages 1-102 (August 2022)
-
Volume 66
Pages 1-112 (July 2022)
-
Volume 65
Pages 1-138 (June 2022)
-
Volume 64
Pages 1-186 (May 2022)
-
Volume 63
Pages 1-124 (April 2022)
-
Volume 62
Pages 1-104 (March 2022)
-
Volume 61
Pages 1-120 (February 2022)
-
Volume 60
Pages 1-124 (January 2022)
-
Volume 71
- Volumes 54-59 (2021)
- Volumes 48-53 (2020)
- Volumes 42-47 (2019)
- Volumes 36-41 (2018)
- Volumes 30-35 (2017)
- Volumes 24-29 (2016)
- Volumes 18-23 (2015)
- Volumes 12-17 (2014)
- Volume 11 (2013)
- Volume 10 (2012)
- Volume 9 (2011)
- Volume 8 (2010)
- Volume 7 (2009)
- Volume 6 (2008)
- Volume 5 (2007)
- Volume 4 (2006)
- Volume 3 (2005)
- Volume 2 (2004)
- Volume 1 (2003)
• Concept for non-contact multi point thermometry in non-transparent packed beds.
• Particles coated with thermographic phosphors to measure temperatures inside the bed.
• Separation of signal from multiple spheres using linear regression and ray tracing simulations.
• Validation of 3-point measurements in regular bed against thermocouple readings.
• Multi-point measurements used to refine heat transfer model of the whole bed.
Packed bed reactors are commonly found in the process industry, for example in flame-assisted calcination for cement production. Understanding the heat transfer inside the bed is essential for process control, product quality and energy efficiency. Here we propose a technique to determine the internal temperature distribution of packed beds based on a combination of lifetime-based phosphor thermometry, ray tracing simulations, and assimilation of temperature data using finite element heat transfer simulations. To establish and validate the technique, we considered a reproducible regular packing of 6 mm diameter aluminum spheres, with one of the spheres in the top layer being electrically heated. If a sphere inside the packing is coated with thermographic phosphors and excitation light is directed towards the packing, luminescence from the coated sphere exits the packed bed after multiple reflection and the sphere's temperature can be determined. Isothermal measurements showed that the temperature obtained by phosphor thermometry is independent of the luminescent sphere location. When imaging the luminescence on a camera, the luminescence distribution in recorded image depended, however, on the position of the sphere. Therefore, in setups with multiple phosphor-coated spheres, their signals can be separated using a least squares fit. We demonstrate the approach using a setup with three luminescent spheres and validated the temperature readings against thermocouple measurements. To obtain the spatial signatures for individual sphere positions required for the least squares fit, ray tracing simulations were used. These provide an efficient alternative to single sphere measurements that are only practical for regular spherical packed beds. Multi-point measurements were used as input to a finite element heat transfer simulations to determine parameters such as particle-to-particle air gap distance. With these, the full temperature distribution inside the bed could be assimilated from the measured values.
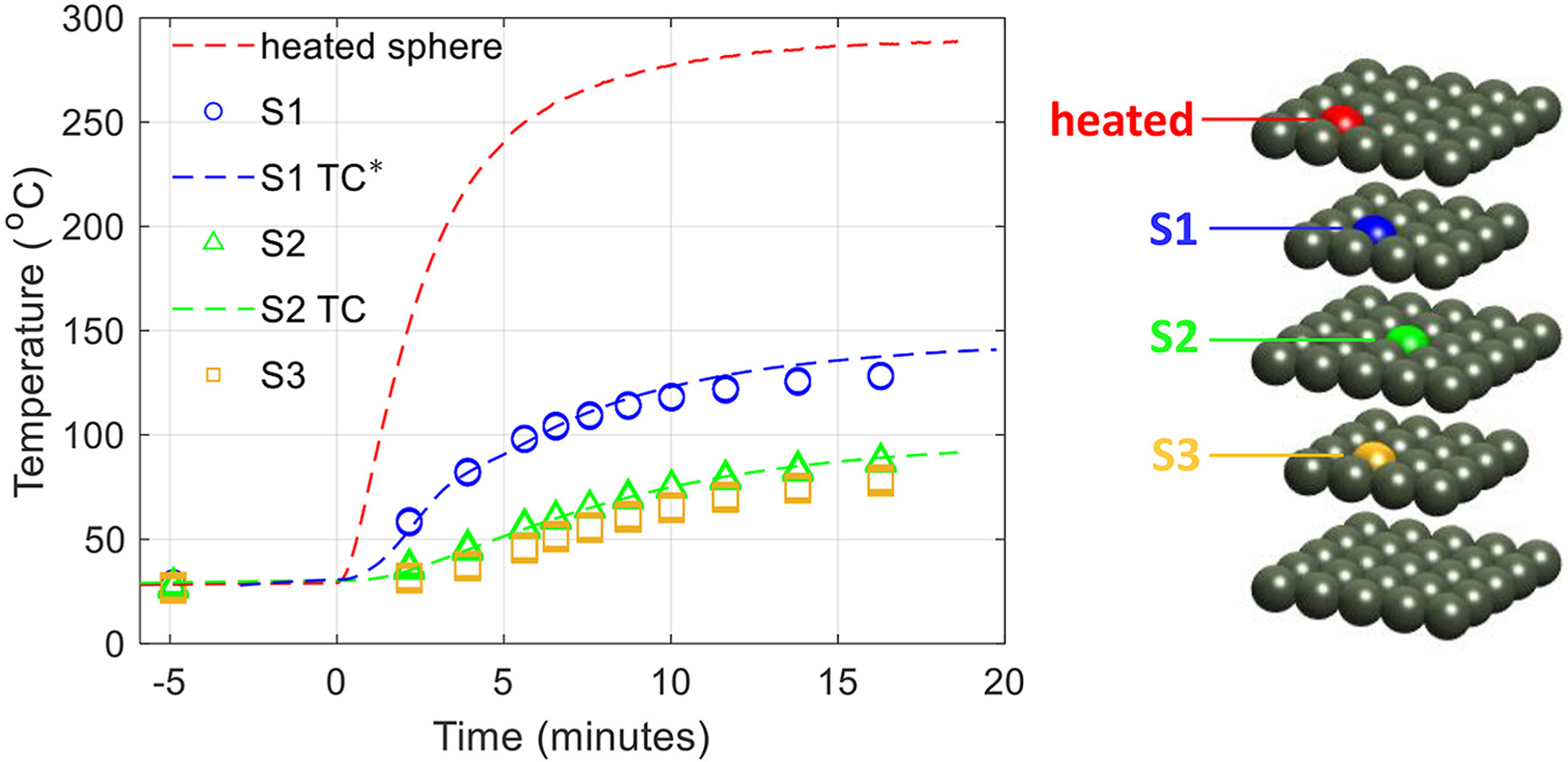