- Volumes 96-107 (2025)
-
Volumes 84-95 (2024)
-
Volume 95
Pages 1-392 (December 2024)
-
Volume 94
Pages 1-400 (November 2024)
-
Volume 93
Pages 1-376 (October 2024)
-
Volume 92
Pages 1-316 (September 2024)
-
Volume 91
Pages 1-378 (August 2024)
-
Volume 90
Pages 1-580 (July 2024)
-
Volume 89
Pages 1-278 (June 2024)
-
Volume 88
Pages 1-350 (May 2024)
-
Volume 87
Pages 1-338 (April 2024)
-
Volume 86
Pages 1-312 (March 2024)
-
Volume 85
Pages 1-334 (February 2024)
-
Volume 84
Pages 1-308 (January 2024)
-
Volume 95
-
Volumes 72-83 (2023)
-
Volume 83
Pages 1-258 (December 2023)
-
Volume 82
Pages 1-204 (November 2023)
-
Volume 81
Pages 1-188 (October 2023)
-
Volume 80
Pages 1-202 (September 2023)
-
Volume 79
Pages 1-172 (August 2023)
-
Volume 78
Pages 1-146 (July 2023)
-
Volume 77
Pages 1-152 (June 2023)
-
Volume 76
Pages 1-176 (May 2023)
-
Volume 75
Pages 1-228 (April 2023)
-
Volume 74
Pages 1-200 (March 2023)
-
Volume 73
Pages 1-138 (February 2023)
-
Volume 72
Pages 1-144 (January 2023)
-
Volume 83
-
Volumes 60-71 (2022)
-
Volume 71
Pages 1-108 (December 2022)
-
Volume 70
Pages 1-106 (November 2022)
-
Volume 69
Pages 1-122 (October 2022)
-
Volume 68
Pages 1-124 (September 2022)
-
Volume 67
Pages 1-102 (August 2022)
-
Volume 66
Pages 1-112 (July 2022)
-
Volume 65
Pages 1-138 (June 2022)
-
Volume 64
Pages 1-186 (May 2022)
-
Volume 63
Pages 1-124 (April 2022)
-
Volume 62
Pages 1-104 (March 2022)
-
Volume 61
Pages 1-120 (February 2022)
-
Volume 60
Pages 1-124 (January 2022)
-
Volume 71
- Volumes 54-59 (2021)
- Volumes 48-53 (2020)
- Volumes 42-47 (2019)
- Volumes 36-41 (2018)
- Volumes 30-35 (2017)
- Volumes 24-29 (2016)
- Volumes 18-23 (2015)
- Volumes 12-17 (2014)
- Volume 11 (2013)
- Volume 10 (2012)
- Volume 9 (2011)
- Volume 8 (2010)
- Volume 7 (2009)
- Volume 6 (2008)
- Volume 5 (2007)
- Volume 4 (2006)
- Volume 3 (2005)
- Volume 2 (2004)
- Volume 1 (2003)
b Key Laboratory of Quantum Physics and Photonic Quantum Information, Ministry of Education, University of Electronic Science and Technology of China, Chengdu, 611731, China
• Regulatory mechanism of (poly)sulfide–oxide interactions by Cu valence states was systematically unraveled.
• Cu2+ with a higher valence state was identified to indirectly regulate the Li bond strength with O2−.
• CuO with stronger Li bonds exhibited higher catalytic activity for the sulfur reduction reactions than Cu2O.
• CuO-based catalytic layer further improved Li–S battery performance at kinetically limited conditions.
Valence state is identified as a key property of transition metal-based catalysts in conventional heterogeneous catalysis research. For a specific monometal element, however, the regulatory role of valence state has seldom explored in emerging energy catalytic applications such as rechargeable lithium–sulfur batteries suffering from sluggish sulfur cathode conversion kinetics. In this study, two monometal oxides with distinct valence states, cupric oxide (CuO) and cuprous oxide (Cu2O), were investigated, revealing valence-state-dependent interactions between oxides and sulfur species, as well as the modulated sulfur reduction reaction (SRR) kinetics. In addition to the inherent Cu2+-enabled surface (poly)thiosulfate redox, divalent Cu2+ and monovalent Cu+ were found to steer the oxygen reactivity and so indirectly tune the lithium bond strength that dictates the surface chemisorption of lithium (poly)sulfides. The stronger interactions between CuO and sulfur species promoted SRR conversion kinetics, enabling enhanced lithium–sulfur battery performance under kinetically demanding conditions such as high-rate capability at 2 C with a moderate sulfur loading of 1.3 mg cm−2 and cycling stability for over 110 cycles at a high sulfur loading of 4.8 mg cm−2. This work is expected to expand the scope of metal-valence-state effect on heterogeneous catalysis and offer an unconventional “indirect” way to regulate lithium-bond chemistry for battery research.
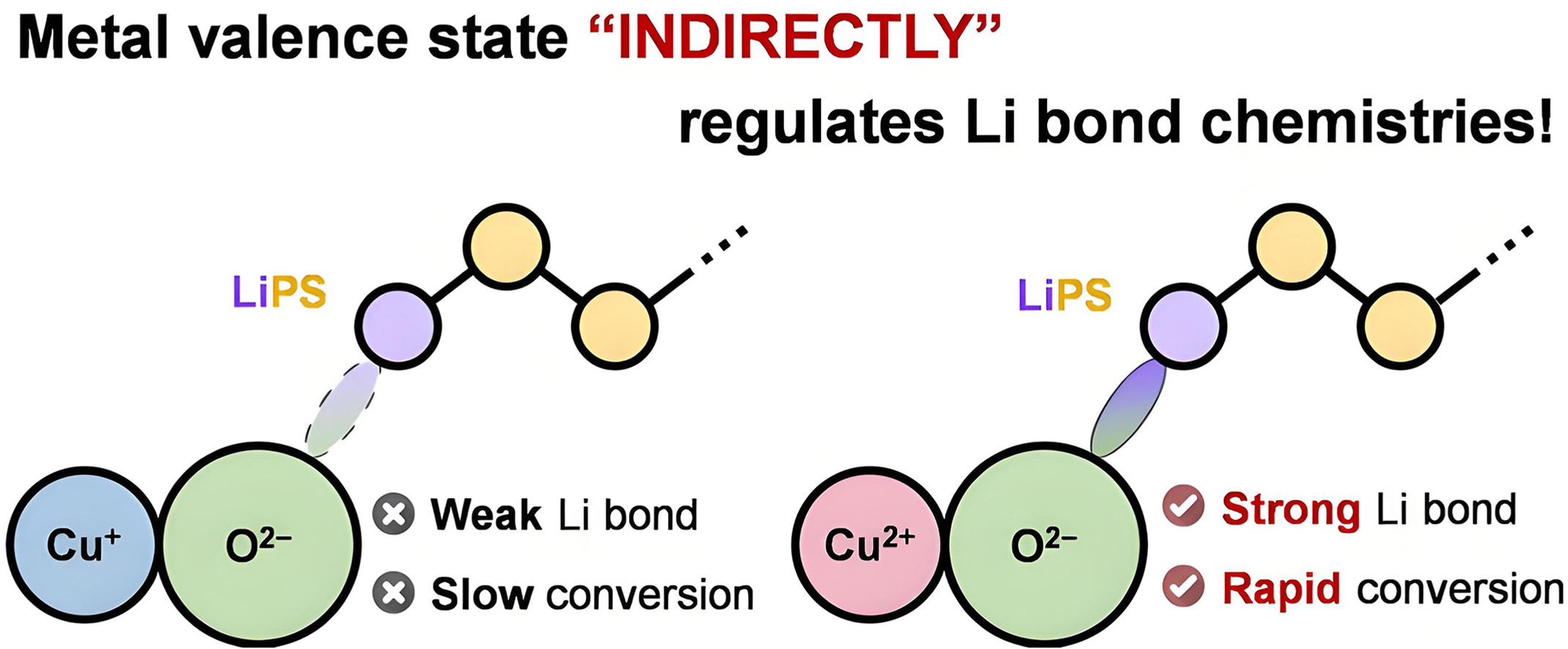